Introduction
Several paths exist toward the realization of photovoltaic devices with efficiency greater than 50%, including multiple junction solar cells (tandems), solar cells using optical frequency shifting (such as up/down conversion or thermophotonics), multiple exciton generation (MEG) from a single photon, multiple energy level solar cells (such as intermediate band solar cells), and hot carrier solar cells. In addition to high efficiency, such cells must also be low in cost, made of polycrystalline thin films grown on inexpensive substrates.
Multiple Junction Solar Cells
Multiple junction solar cells, or tandem solar cells, consist of multiple, single-junction solar cells joined together or stacked upon each other, with each solar cell absorbing the part of the solar spectrum closest to its band gap. Existing tandem devices have achieved efficiencies over 37% at a concentration of 173 suns, and further efficiency increases can be achieved by increasing the number of different junctions. Despite the high efficiency potential, tandem devices experience a fundamental limitation relating to the availability of materials that simultaneously allow high efficiency through low defect densities and the choice of optimal band gaps. In addition to fundamental advances in understanding defects and recombination, exploring new materials and nanostructures may also revolutionize multiple junction devices by allowing control over band structure, growth, and defects.
Optical Frequency Shifting
Optical frequency shifting cells involve the transformation of the solar spectrum from one with a broad range of energies to one with the same power density but a narrow range of photon energies (see Figure 1). One central feature of these approaches, which include up and downconversion (i.e., creating a single high-energy photon from two lower-energy photons or creating two lower-energy photons from a single higherenergy photon, respectively) and thermophotonics (i.e., using the refrigerating action of an ideal light emitting diode to increase the emitted photon energy), is that the transformation of the solar spectrum is done separately with a material that is not part of the solar generator, thus increasing the efficiency of an existing solar generator structure via additional coatings or external elements. There are several fundamental challenges in these approaches, including demonstration of cooling due to optical emissions, as well as more efficient processes for up-conversion.
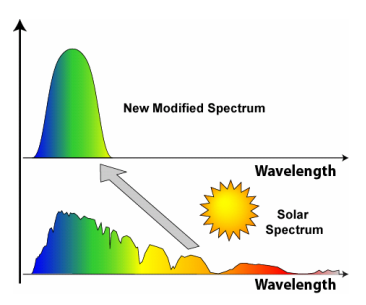
Multiple Exciton Generation Solar Cells
A central limitation of existing solar cell approaches is the one-to-one relationship between an absorbed photon and a generated electron-hole pair. The process of impact ionization, known for decades in bulk semiconductor crystals, allows the conversion of single high-energy photons to multiple electron-hole pairs, but with relatively low efficiency. Recent experimental reports of multiple exciton generation (MEG) in nano-sized (quantum dot) semiconductors indicate much more efficient generation of multiple electron-hole pairs compared to bulk materials (see Figure 2). For example, semiconductor quantum dots of PbSe and PbS have demonstrated high efficiencies of multiple exciton generation, producing as many as three excitons per absorbed photon. While the basic physical phenomenon has been demonstrated, additional challenges remain, including efficient transfer and extraction of the generated charges from the nano-structured materials.
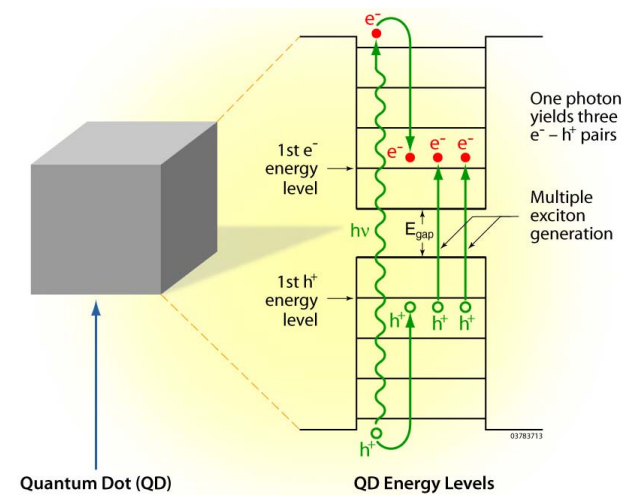
Figure 2 Multiple exciton generation in quantum dots. Because of quantum confinement in the small nanoscale semiconductor QD particle, the energy levels for electrons and holes are discrete. This slows hot exciton cooling and enhances multiple exciton formation. A single absorbed photon that has an energy at least 3 times the energy difference between the first energy levels for electrons and holes in the QD can create 3 excitons. The bandgap of the bulk semiconductor is indicated as Eg.
Multiple Energy Level Solar Cells
In multiple energy level solar cells, the mismatch between the incident energy of the solar spectrum and a single band gap is accommodated by introducing additional energy levels such that photons of different energies can be efficiently absorbed. Multiple energy level solar cells can be implemented either as localized energy levels (first suggested as a quantum well solar cell) or as continuous mini-bands (also called intermediate band for the first solar cell to suggest this approach). Both cases, which are shown in Figure 3, have a fundamental similarity in that the key issue is the generation of multiple light-generated energy levels for electrons.
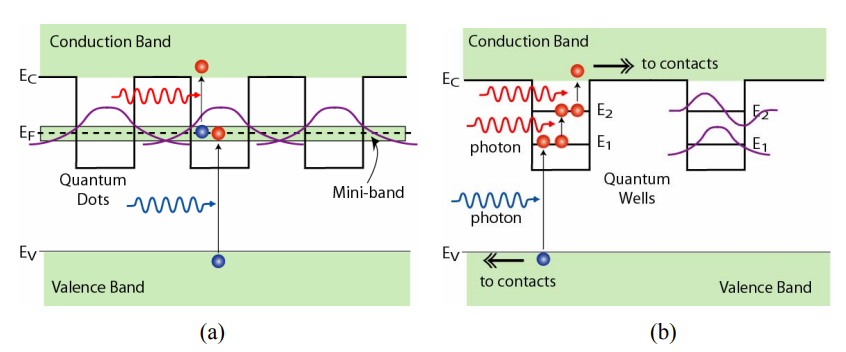
Hot Carrier Solar Cells
Hot carrier solar cells utilize selective energy contacts to extract light generated hot carriers (electrons and holes) from the semiconductor regions before they have thermalized with the semiconductor lattice (i.e., converted their excess energy to heat). This allows higher efficiency devices (up to a thermodynamic limit of 66% at one sun intensity) by reducing the thermalization (heat) losses in single-junction solar cells. To benefit from this approach, the escape of the hot carriers through the energy-selective contacts must be faster than the various inelastic scattering processes that lead eventually to thermalization to the lattice temperature as shown in Figure 4. Specific materials, in particular, materials with low dimensions such as quantum dots, show slowed carrier cooling and thus hold the promise for realizing such hot carrier solar cells. The obvious prerequisite is reducing the cooling rate well below the hot carrier collection rate and developing the energy-selective contacts for hot carrier collection.
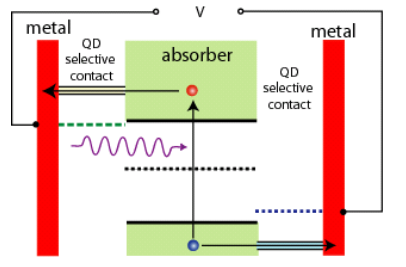